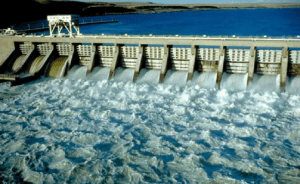
Hydropower requires stored water
A. Introduction
Commonly known as hydropower, hydroelectric power is among the first and most accepted forms of renewable energy. It uses the energy of falling or flowing water to create electricity, therefore offering a dependable and sustainable power supply.
Because hydropower depends on the natural water cycle for fuel and generates minimal greenhouse gas emissions, it is regarded as a clean energy source. This makes it crucial for several countries trying to reduce their dependency on fossil fuels and fight climate change.
We shall explore the fundamental ideas of hydroelectric power generation, its scientific background, and the other technical procedures allowing its operation in this blog article.
B. The Basics of Hydropower
1. What is hydropower?
Hydropower is the generation of electrical power from flowing or falling water. Usually, it entails the transformation of water’s kinetic or potential energy into mechanical energy by means of turbines and generators, subsequently into electrical energy. Because of its sustainability, low environmental impact, and capacity to offer a constant supply of electricity, this energy source is quite preferred.
2. Brief History of Hydropower
Long before contemporary energy first emerged, in ancient civilizations, is where hydropower began. Early uses concentrated on water wheels for grinding grains and other mechanical chores. In ancient Greece in the third century BCE, a waterwheel was first documented being used.
The industrial revolution was a major turning point since developments in turbine technology enabled water power generate electricity. The first hydroelectric power plant in the world started running in Appleton, Wisconsin, USA in 1882; since then, the technology has developed significantly and is now a pillar of contemporary energy architecture.
3. The Concept of Energy Conversion:
The basic concept of energy conversion is: potential energy to kinetic energy to electrical energy. Hydropower generates electricity by harnessing the energy of moving water.
To summarize the process succinctly:
- Water is stored in a reservoir (potential energy).
- Water is released and flows down (conversion to kinetic energy).
- Flowing water spins a turbine (conversion to mechanical energy).
- The turbine drives a generator, producing electricity (conversion to electrical energy).
- Electricity is transformed and distributed for use.
The entire process relies on the continuous and renewable nature of the water cycle, making hydropower a sustainable energy source.
C. Hydrological Cycle and Hydroelectric power
In order to understand hydroelectric power generation comprehensively, it’s crucial to delve into the hydrological cycle, various factors affecting water availability, and the significance of river basins. These elements are integral to the efficiency and sustainability of hydroelectric power systems.
1. Pivotal Role of the Water Cycle: In-depth Look
The hydrological cycle—also called the water cycle—is the constant circulation of water on, above, and below Earth’s surface. Ensuring a sustained supply of water for the development of hydroelectric electricity depends much on this natural process. Important phases of the water cycle consist in:
- Evaporation: Solar energy heats bodies of water such as lakes, rivers, and oceans, causing water to evaporate and transform into water vapor, which rises into the atmosphere.
- Transpiration: Plants absorb water through their roots and release it into the atmosphere as vapor through small pores (stomata) in their leaves.
- Condensation: As water vapor rises and cools, it condenses to form clouds. This step is crucial for the accumulation of moisture in the atmosphere.
- Precipitation: When clouds become saturated, water falls back to the Earth’s surface in the form of rain, snow, sleet, or hail. This replenishes rivers, lakes, and reservoirs.
- Runoff: Water from precipitation flows across the land’s surface, contributing to rivers and streams that eventually feed into reservoirs or return to the ocean.
- Infiltration and Percolation: Some of the precipitation infiltrates the soil, recharging groundwater supplies. This groundwater can later feed into rivers and lakes, maintaining their levels.
2. Factors Affecting Water Availability for Hydropower
Several factors influence the availability and sustainability of water resources for hydroelectric power generation:
- Precipitation: The amount and frequency of precipitation directly impact the volume of water available for hydroelectric plants. Regions with high rainfall or snowfall are ideal for hydropower, as they provide a reliable inflow of water.
- Evaporation: Higher temperatures and prolonged sunlight can increase evaporation rates, reducing the amount of water available for hydroelectric power. This factor can be particularly critical in arid or semi-arid regions.
- Runoff: The rate of runoff depends on the terrain, soil characteristics, vegetation cover, and rainfall intensity. Effective runoff management ensures that more water reaches reservoirs and is available for power generation.
- Seasonal Variations: Seasonal changes, such as monsoons or dry spells, can cause fluctuations in water availability. Hydropower systems need to account for these variations to maintain consistent energy production.
- Climate Change: Long-term changes in climate patterns can significantly affect water availability through altered precipitation, increased evaporation, and changes in snowmelt timing. These factors necessitate adaptive management strategies for hydropower plants.
3. River Basins and Their Importance for Hydropower
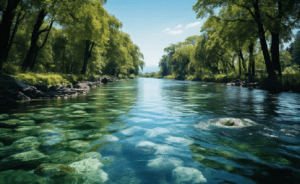
River basins, which drain land areas through a river and its tributaries, play a fundamental role in the success of hydroelectric projects. Key points include:
- Water Collection: River basins gather and channel water from precipitation and tributaries into a central river system, ensuring a steady supply of water for reservoirs.
- Storage and Regulation: Dams and reservoirs within river basins store water and regulate its flow, enabling controlled release for power generation, especially during peak demand periods or dry seasons.
- Ecological Considerations: Healthy river basins support diverse ecosystems and provide ecological services such as water purification and habitat for aquatic life. Sustainable hydropower projects must consider the environmental impacts on river basins and strive to minimize disruption to local ecosystems.
- Watershed Management: Effective management practices within river basins, like afforestation, soil conservation, and sustainable agriculture, improve water retention and reduce sedimentation, enhancing the efficiency and longevity of hydropower infrastructure.
Examining the hydrological cycle, the several elements influencing water availability, and the vital function of river basins helps us to better grasp how hydropower systems run and their significance in the scene of world energy. Development and best optimization of sustainable hydroelectric power generating projects depend on this realization.
D. Scientific Concepts in Hydroelectricity
E. Hydraulics and Hydropower
The efficiency and effectiveness of hydroelectric power generating mostly depend on ideas developed from fluid mechanics and hydraulics. This chapter will investigate these basic ideas, delve into the ideas of water pressure and flow rate, clarify the relevance of “head” and “discharge,” and underline how hydraulic efficiency is absolutely essential in maximizing hydropower plants.
1. Basic Principles of Fluid Mechanics
Fluid mechanics is the study of fluids (liquids and gases) and the forces acting on them. In the context of hydropower, it involves understanding the behavior of water as it flows and interacts with hydraulic structures. Key principles include:
- Continuity Equation: This principle states that the mass flow rate of water is constant along a streamline. For incompressible fluids like water, the equation is expressed as (A1V1 = A2V2), where (A) is the cross-sectional area and (V) is the flow velocity. This helps in calculating how water speed changes as it moves through different sections of the hydropower system.
- Bernoulli’s Principle: This states that an increase in the speed of a fluid occurs simultaneously with a decrease in pressure or potential energy. Bernoulli’s equation is crucial for understanding energy changes in water as it moves through the hydropower system, including how pressure and velocity interplay.
- Navier-Stokes Equations: These equations describe how various forces influence the velocity field of a fluid substance.
In hydropower, these complex equations are simplified for different applications to model the motion of water and optimize design parameters.
2. Water Pressure and Flow Rate
Water pressure and flow rate are foundational concepts in hydraulics, determining how effectively a hydropower system can operate:
- Water pressure The force exerted by water per unit area. It’s influenced by the height of the water column (hydraulic head) and the density of water. Higher water pressure means more potential energy is available for conversion into kinetic and, subsequently, electrical energy.
- Flow Rate: This refers to the volume of water passing a point per unit of time, typically measured in cubic meters per second (m³/s). The flow rate determines how much kinetic energy is available to drive the turbines and affects the overall power output.
3. Head and Discharge: Key Concepts in Hydropower
- Head: The height difference between the water source and the turbine, also known as the hydraulic head. It’s a measure of potential energy. Higher head means more potential energy converted into kinetic energy as the water falls, leading to higher electricity generation efficiency. Head can be classified into:
- Gross Head: The total height difference.
- Net Head: Gross head minus losses due to friction and turbulence in the system.
- Discharge: The volume of water flowing through the hydropower system per unit of time, also known as flow rate. Discharge is a crucial factor in determining the capacity of a hydropower plant. It’s also used to calculate the potential electrical power generation using the formula: [ P = \eta \rho g Q H ]
Where (P) is the power, (eta) is the hydraulic efficiency, (rho) is the density of water, ( g ) is the acceleration due to gravity, ( Q ) is the discharge (flow rate), and ( H ) is the net head.
4. Hydraulic Efficiency in Hydropower Systems
A measurement of how well the hydropower system turns water’s potential energy into mechanical energy in the turbine and, hence, into electrical energy is hydraulic efficiency. Elements affecting hydraulic efficiency include:
- Turbine Design: The shape and type of turbine blades can significantly affect how efficiently water energy is converted into mechanical energy. Common types include Pelton, Francis, and Kaplan turbines, each suited for different head and flow conditions.
- Friction Losses: As water flows through pipes, penstocks, and turbines, friction between the water and surfaces can cause energy losses. Minimizing these losses involves using smooth, optimized channel designs and proper maintenance.
- Turbulence: Turbulent flows can lead to energy dissipation and decrease hydraulic efficiency. Ensuring laminar flow through careful engineering design minimizes turbulence.
- Operational Practices: Optimal operation and maintenance of hydropower systems ensure that components like turbines and generators run efficiently, reducing downtime and maximizing energy output.
Understanding the basic ideas of fluid mechanics, the function of water pressure and flow rate, the important concepts of head and discharge, and the elements influencing hydraulic efficiency will help us to better value the complex science supporting the generation of hydroelectric power. Design, running, and optimizing hydropower depends on these ideas.
F. Types of Hydroelectric Power Plants
There are several types of hydroelectric power plants, each with unique characteristics and applications:
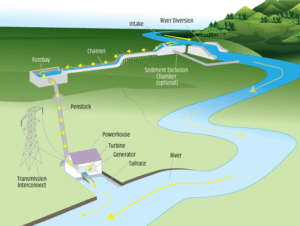
- Run-of-the-River Plants: These plants utilize the natural flow of rivers without significant storage. They are advantageous for their minimal environmental impact but can be less reliable due to water flow variability.
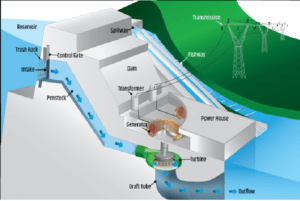
- Storage (Reservoir) Plants: These plants store large volumes of water in reservoirs created by dam construction. This stored water can be released as needed to generate electricity, offering reliability and flexibility in energy production.
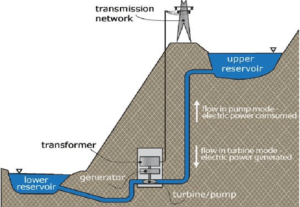
- Pumped-Storage Plants: These plants function by pumping water from a lower reservoir to a higher one during periods of low electricity demand. During peak demand, the stored water is released to generate electricity. This method helps balance supply and demand and supports smart grid integration.
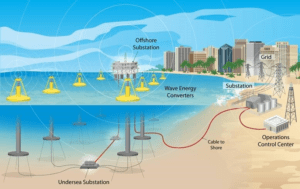
- Offshore Hydropower: Emerging technologies aim to harness the power of ocean currents, tides, and waves to generate electricity. While still in development, offshore hydropower holds potential for significant energy production with minimal land use.
G. Power Plant Systems
Hydroelectric power plants are complex systems that integrate various components and technologies to efficiently convert the energy of water into electrical power. This chapter explores the key components of a hydropower plant, the role of control systems and automation, and the environmental considerations in power plant design.
1. Components of a Hydropower Plant
A typical hydropower plant consists of the following major components:
- Intake: The structure that diverts water from the river or reservoir into the power plant. It is designed to regulate the flow of water and prevent debris from entering the system.
- Penstock: The large, high-pressure pipe that carries the water from the intake to the turbine. The penstock is engineered to withstand the significant water pressure and minimize energy losses.
- Turbine: The heart of the hydropower plant, where the kinetic energy of the water is converted into mechanical energy. As discussed in the previous chapter, different turbine types (Pelton, Francis, and Kaplan) are used depending on the site’s head and flow characteristics.
- Generator: The electromechanical device that converts the mechanical energy from the turbine into electrical energy. Generators are designed to maintain a stable output voltage and frequency.
- Transformer: This component steps up the generator’s output voltage to the appropriate level for transmission through the electrical grid.
The integration and optimization of these components are crucial for the overall efficiency and performance of the hydropower plant.
1.1 How is the mechanical energy used?
-
Turbines: The force of flowing or falling water striking the turbine blades produces mechanical energy, causing them to spin. This rotational movement represents the mechanical energy harnessed from the water.
-
Generator: Connected to the turbine, the generator consists of coils of wire rotating within a magnetic field. As the turbine turns, it rotates the coils within the magnetic field, inducing an electric current in the wires.
-
Electrical Energy: The mechanical energy from the spinning turbines is thus transformed into electrical energy within the generator. This electrical energy, in the form of alternating current (AC), is then transmitted through power lines to homes, businesses, and industries for various applications, such as powering appliances, lighting, machinery, and more.
Overall, hydropower generates mechanical energy to rotate generators, which then convert this mechanical energy into electrical energy. This electrical energy is essential for meeting the power needs of communities and industries, contributing to the functioning of modern society and various economic activities.
2. Control Systems and Automation
Hydropower plants employ advanced control systems and automation technologies to ensure efficient, reliable, and safe operation. Key aspects include:
- Supervisory Control and Data Acquisition (SCADA): SCADA systems monitor and control the various plant components, allowing operators to remotely manage the plant’s operations and respond to changing conditions.
- Automated Turbine and Generator Control: Sophisticated control algorithms optimize the operation of turbines and generators, adjusting parameters like gate opening, blade pitch, and excitation to maximize efficiency and power output.
- Grid Integration and Synchronization: Control systems ensure that the generated electricity is properly synchronized with the grid in terms of voltage, frequency, and phase, enabling seamless integration.
- Safety and Emergency Systems: Automated systems monitor critical parameters and initiate emergency shutdown procedures to protect the plant and its personnel in the event of malfunctions or natural disasters.
3. Environmental Considerations in Power Plant Design
Hydropower projects have a significant impact on the surrounding environment, and their design must address these considerations:
- Ecological Impacts: Hydropower plants can disrupt aquatic ecosystems, affect fish migration, and alter the natural flow of rivers. Mitigation measures, such as fish ladders and environmental flow releases, are implemented to minimize these impacts.
- Water Quality Management: Hydropower reservoirs can experience water quality issues like thermal stratification, eutrophication, and sedimentation. Plant design and operational practices aim to maintain water quality for both power generation and downstream uses.
- Greenhouse Gas Emissions: While hydropower is generally considered a clean energy source, the construction and operation of dams and reservoirs can lead to the release of greenhouse gases, particularly methane. Sustainable design and management practices help minimize these emissions.
- Landscape and Land Use: Hydropower projects often involve the creation of large reservoirs, which can displace communities, submerge valuable land, and alter the local landscape. Careful planning and stakeholder engagement are crucial to addressing these issues.
By understanding the key components of a hydropower plant, the role of control systems and automation, and the environmental considerations in power plant design, we can appreciate the technological sophistication and multifaceted nature of hydroelectric power generation. These elements are essential for the development, operation, and sustainability of hydropower projects.
H. Future of Hydropower
1. Advances in Hydropower Technology Ongoing technological advancements are enhancing the efficiency, flexibility, and environmental performance of hydropower systems:
- Improved turbine and generator designs to increase energy conversion rates.
- Digitalization and automation to optimize plant operations and maintenance.
- Pumped-storage hydropower systems with advanced reversible turbine-generator units.
- Innovative solutions for small-scale and run-of-river hydropower projects.
2. Small-scale Hydropower and microhydro The development of small-scale and micro-hydropower projects is gaining momentum, offering several benefits:
- Smaller environmental footprint and reduced social impacts compared to large-scale projects.
- Increased accessibility and distributed generation, especially in remote or off-grid areas.
- Potential for integration with other distributed energy resources, such as solar and battery storage.
Simplywall This is really interesting, You’re a very skilled blogger. I’ve joined your feed and look forward to seeking more of your magnificent post. Also, I’ve shared your site in my social networks!
This is lovely. Thanks a lot. Your comment elevated my enthusiasm.
Mountsinai Pretty! This has been a really wonderful post. Many thanks for providing these details.
Your point of view caught my eye and was very interesting. Thanks. I have a question for you.